Teamwork Pays Off
California wastewater treatment plant saves fuel costs by using waste heat from a nearby power plant to dry biosolids
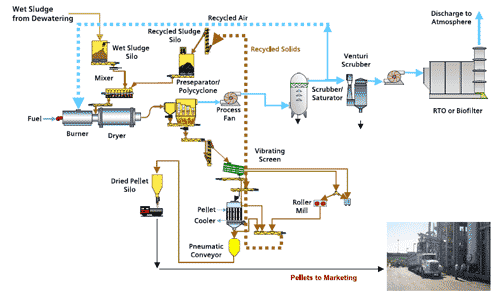
With an innovative design that integrates a state-of-the-art biosolids drying facility with the heat from a combined-cycle cogeneration plant, Corona, Calif.’s Clearwater Wastewater Treatment Plant No. 1 is helping to save energy.
The 15.5 million gallon per day (MGD) (59 million liter per day) wastewater facility is using a directly heated biosolids dryer to process 110 wet tons per day (9,167 pounds per hour) of municipal biosolids, with a dry solids content of 16 percent. What makes this project even more unique is the use of waste heat from the city’s first power plant to preheat the dryer’s combustion air and to supply the low-grade heat required to promote biological growth in the wastewater treatment plant’s digesters. Using a dual fuel source of natural gas and digester gas, the energy-efficient biosolids dryer produces an end product that complies with the U.S. Environmental Protection Agency 503 Part 40 rule under the Clean Water Act for heat-dried Class A biosolids.
The power plant, constructed at the same time as the biosolids dryer facility, is a 31-megawatt (MW) combined-cycle cogeneration facility with more than enough electricity to operate the entire wastewater treatment facility and provide 500 º Fahrenheit (468ºC) of waste heat to the biosolids dryer.
Background
In 2001, around the time of California’s power shortages and increasing energy costs, the city of Corona decided to build a 30MW cogeneration power plant at the site of its largest wastewater treatment plant. The power plant would ensure a reliable supply of electricity at stable and predictable prices to the city’s businesses and surrounding residential areas. The city also decided that the wastewater treatment plant should begin producing Class A biosolids because local counties were severely limiting the land application of Class B biosolids, and the city was forced to truck its Class B biosolids greater distances for disposal, costing more money.
The city established a municipally owned electric utility and developed an agreement with Southern California Edison whereby the city would provide electric retail services as an electric services provider (ESP). Building the power plant at the wastewater treatment facility’s site made sense for several reasons:
- The reclaimed water produced by the wastewater plant could be used as cooling water for the power generation facility.
- The waste heat from the power plant could be beneficially used by the wastewater plant’s digesters.
- The waste heat could also be beneficially used in a Class A biosolids process.
- The combined facility operation would be more cost-effective by using the same personnel to operate both systems.
- Construction costs would be reduced.
Headline: The Wastewater Treatment Process
An activated sludge process treats the wastewater at plant No. 1. First, large solids are removed by bar screens, and abrasive materials, known as grit, are removed from the flow stream to protect downstream equipment. Remaining inert solids are removed in primary clarifiers and, after biological treatment, the mixture of the “bugs” and wastewater, known as mixed liquor, is discharged into secondary clarifiers to separate the solids from the effluent. The treated effluent is reclaimed and used as beneficial irrigation water in local Butterfield Park. The treated effluent that is not reclaimed is discharged to Temescal Creek or sent to percolation ponds for recharging underground aquifers.
Biosolids generated during the treatment process are removed from the flow stream, treated in digesters and thickened by a gravity belt thickener to 4 or 5 percent solids. The biosolids are then sent to the belt filter presses and mechanically dewatered to between 13 and 16 percent dry solids. Before the biosolids dryer was installed, the dewatered, Class B biosolids were trucked off site by a contract hauler for disposal.
Now, a Sernagiotto convective thermal dryer (CTD) from Siemens Water Technologies heat dries the biosolids cake, which results in a 4-to-1 volume reduction and a beneficial reuse material. The city may sell the Class A biosolids as a soil amendment or as an organic fuel to local industry. The tested heat value of the dried product is similar to a low-grade coal – around 6,500 Btu’s per pound of product. |
Integrating Power Generation and Biosolids Drying
After evaluating different processes that could produce Class A biosolids using heat generated by the power generation facility, the city chose a biosolids heat dryer. The evaluation showed that optimum use of heat for the dryer was 93,000 pounds per hour at 500 to 540° Fahrenheit (468 to 508°C), an amount that would not affect the heat dryer’s equipment sizing and would only reduce power generation by 0.1 MW. The city would be able to use the digester gas to fuel the burner for the biosolids dryer, which would save the city an extra 2.3 million Btu’s per hour.
According to West Curry, wastewater treatment plant manager, the biosolids dryer was expected to reduce the normal wet cake handling to 20 percent of the present production volume, saving the city money. It would also prevent odors that would have been a major problem because of the plant’s location within city limits. The city planned to sell all of the effluent water produced and beneficially reuse the Class A biosolids for land applications. In turn, the community would use the produced electricity and the city would sell any excess to the electrical grid.
The city teamed up with engineering firm CH2M Hill to provide project development and a detailed design for integrating the power plant with a biosolids drying facility.
The Convective Thermal Dryer Process
The biosolids dryer system, which operates 24 hours a day, six days a week, evaporates water from the wet cake produced by the belt filter press dewatering system. The sludge provided by the belt presses contains 13 to 16 percent solids by weight at temperatures of 55 to 95º Fahrenheit (23 to 63ºC). The incoming sludge is fed via a screw conveyor from the belt press area to a wet sludge storage silo designed to hold two hours’ capacity (270 cubic feet; 7.6 cubic meters). A dosing hopper is supplied at the silo’s exit to control the feed of wet sludge being transferred to a mixing chamber. The mixing chamber is a commercial paddle-style mixer that combines wet sludge with previously dried pellets fed from the recycling product silo to form a mixture of approximately 70 percent solids content to be fed to the drying drum. The mixed sludge is introduced into a hot gas stream prior to the rotary drum that consists of fuel combustion air and recycled process air. The hot gas stream operates between 700 and 800º Fahrenheit (668 and 768°C). This process requires a maximum of 13 million British thermal units per hour (Btu/hr) thermal energy input. The hot gas stream is produced by the combustion of natural gas as the main energy source and digester gas as a secondary energy source. When available, the digester gas reduces the consumption of the natural gas input by 2.3 million Btu/hr.
A heat exchanger installed in the recycle process air stream transfers 6 million Btu/hr of the waste heat produced by the cogeneration plant, reducing the total fuel consumption. The natural gas burner fuel consumption is automatically controlled by redundant temperature monitors on the dryer system. If gas temperatures reach a high level, an automatic water spray is added to the incoming mixed sludge/gas mixture to rapidly lower the drying drums’ exit temperature. Should the exit temperature continue to rise, a high-volume spray automatically extinguishes any combustion in the dryer.
The hot gas is composed of products of combustion, recycled process air and leakage air. The oxygen content of the gas is kept below 8 percent to prevent fires and explosions. As the pellets pass through the drying drum, they are exposed to the hot gas stream, which evaporates the water. To help the evaporation process, the drying drum is equipped with a three-pass design and internal flights. While the drum rotates at a slow speed, it lifts and mixes the sludge with the hot gas stream, thus increasing the contact area and creating a spherical-shaped pellet.
Air Handling and Dried Product Separation
The mixture of hot gas and dried pellets exits the drying drum and travels through a ceramic-lined duct to the pre-separator and poly-cyclone. The gas stream and pellets enter the pre-separator, where the gas stream is forced to change direction around an impingement plate. The pellets strike the ceramic-lined plate and fall to the bottom hopper for discharge through a rotary valve to be further processed. The gas stream containing entrained dust particles continues on to the poly-cyclone, which contains 24 small cyclone units.
The gas stream enters the poly-cyclone, which spins the gas in a radial direction, forcing the heavy dust particles out of the stream. These particles settle to the bottom hopper for discharge through a rotary valve for further processing.
The gas stream, which contains micron-size particles, continues to the venturi scrubber where the gas is rapidly accelerated through a throat section supplied with plant effluent (reuse) water injected tangentially at the top of the venture scrubber. The water swirls down the throat section, covering the walls to prevent buildup and abrasion. Additional reuse water is introduced in the throat section to guarantee complete coverage of the air stream. The cyclonic separator allows the cleaned gas to exit while collecting the dust-laden water in the bottom reservoir.
The cleaned gas stream is then directed to a scrubber tower, where large cool-water sprays lower the gas temperature to precipitate water picked up in the dryer and venturi while removing small dust particles that have passed through the previous cleaning equipment.
Process Air Distribution and Regenerative Thermal Oxidizer
From the scrubber tower, the gas enters a demister, packed with plastic media to remove as much entrained water as possible before continuing to the process delivery fan. The fan delivers recycled air to the furnace and drying drum while creating a negative pressure that conveys the pellets from the drying drum to the pre-separator. The fan discharges approximately 85 percent of the gas back to the dryer as recycled gas, while exhausting the other 15 percent to the Regenerative Thermal Oxidizer (RTO).
Regenerative Thermal Oxidizer
The RTO oxidizes any volatile organic compounds (VOCs) in the air stream by heating the exhaust gases to 1400º Fahrenheit (1368°C), breaking any molecular bonds of the hydrocarbons to produce carbon dioxide and water. This non-hazardous gas stream is then discharged to the atmosphere through a 65-foot (19.8 meter) stack for better atmospheric dispersion.
Dried Product
The dried product collected in the bottom of the pre-separator and poly-cyclone exits through two rotary valves that create an air lock between this equipment and material transfer equipment. The material transfer equipment consists of two screw conveyors and a bucket elevator that discharge the pellets to a vibrating screener. The screener separates the material into four different sizes: trash – large items, such as plastics over 10mm; oversized material – 4-10mm; product – 1-4mm; fines – less than 1mm. The trash and materials above 10mm are collected in an enclosed trash bin for manual disposal in the treatment plant. The oversized material is directed to a roller mill to be crushed into 1mm or smaller particles. The roller mill is equipped with a magnet to remove metal particles before crushing, to prevent sparking. The crushed material then travels to the recirculation screw for discharge to the recycling product bin.
The 1-4mm product is directed to a pellet cooler designed to reduce the temperature of the dried material from 158 to 90º Fahrenheit (126 to 58°C). The cooled product is then transported to the final product storage silo by a pneumatic transporter.
The final product storage silo can store 1 ¼ days of product-sized dried material until it is loaded into trucks using a screw conveyor and an automated loading chute. The product loading system also includes a mineral oil delivery system to coat the dried material to reduce dust when loading. All dry material handling and storage equipment is equipped with ventilation via a fugitive dust fan system.
Conclusion
Today, Corona’s electric utility is providing electric service from a portfolio of electric power purchases from the market as well as from its cogeneration plant. The utility currently serves loads with about 25MW of peak demand. With the biosolids dryer working in conjunction with the power plant, the city has reduced the volume of biosolids leaving Plant No. 1 by 80 percent, and is producing a Class A beneficial reuse product.
This article originally appeared in the 11/01/2007 issue of Environmental Protection.
About the Author
Joseph H. (Joey) Herndon is USFilter's Dragon Dryer product manager. Based in Thomasville, Ga., he is a 32-year USFilter employee. During his career in the water and wastewater industry, Joey has worked extensively with fertilizer brokers and landowners in the southeastern United States. He can be contacted at (229) 227-8727.