Classroom Vision
Helping environmental-science students see the invisible through instruction and instructional tools
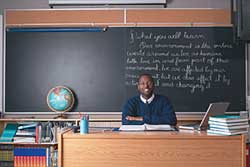
Students enjoy the visible bounty of the Biosphere without a clue of the wondrous invisible world that embraces them. This invisible world indeed sustains their very lives, for within this microcosm exists not only the supply of life-preserving oxygen, but also potentially life-destroying microbes and toxic gases. Engaging students in the enormity and possible trauma of the transparent can be quite a challenge for environmental-science instructors. Nevertheless, this endeavor is critical for advancing the understanding of conditions that make the world both livable and precarious.
In recent years, educational researchers have taken up the challenge of studying students' understanding of invisible physical phenomena. Their studies have included a variety of designs and methods and have been conducted within various frameworks of educational theory. Many studies have included the use of instructional tools. Tool use in education, however, has many nuances, depending on the conceptual framework and instructional approach employed. My definition of "instructional tools" will simply be teaching/learning aids like computer and physical models of invisible phenomena. Classroom lectures or textbook readings will be referred to simply as "instruction."
Manifesting the Invisible with Instructional Tools
A variety of educational research has been undertaken using instruction and instructional tools to discover how students learn to understand invisible conditions, such as the nature and dispersion of air contaminants. In this section, three studies are briefly reviewed to examine student learning with the aid of computer and/or physical models along with specially prepared or more traditional instruction.
Discovering a Familiar Environment
Recently, 30 urban middle school students were evaluated for their understanding of air and pollution particles using student drawings before and after specially prepared instruction. The researchers, Singer, Tal, & Wu (2003), fashioned a project-based science (PBS) curriculum that incorporated a context for the particulate nature of matter concepts; learning technologies (including eChem, a computer-based, professional-quality, visualizing tool); activities that used macroscopic, microscopic/molecular, and symbolic representations across levels to illustrate the targeted concepts; and learning events sequenced in a logical manner to allow the application of student knowledge. Furthermore, the study authors set these four curriculum components within a perspective and a content phase, which the authors referred to as the curriculum's two main enactment phases.
The perspective phase established the context (real-world setting) for the course content, while the content phase provided numerous opportunities for student engagement in content-related activities (the construction of gum-drop models to represent atoms and molecules). Thus, as students observed real-world, familiar situations and engaged in computer and physical modeling, the teachers expected the students would construct a better picture of molecules, both internally and on paper.
After employing their PBS teaching strategy, the researchers found substantial positive improvement in student depictions of air and pollution at the micro-level. More than 90 percent of pre-tested students produced air drawings that were considered macroscopic representations (blank pages for clean air or landscape pictures with clouds, sun, and trees). Whereas, on the post-test, more than 60 percent of the students' air drawings demonstrated that their understanding of atoms and molecules changed substantially. The majority of students illustrated chemical symbols, chemical formulas, or pictorial representations of air and pollution at the microscopic and molecular/symbolic levels. However, it is unclear from the evidence that students' adoption of the word "molecule" was accompanied by an underlying shift in their perception of matter. Nevertheless, to help substantiate findings, five students from one class were interviewed to probe their level of comprehension. All five showed accurate particle arrangement and movement when asked to draw the condition of molecules in the solid, liquid, and vapor states of matter.
Technology-based and Traditional Instruction
The effectiveness of the use of technology-based instruction, along with traditional chemistry instruction to improve students' comprehension of a familiar science principle demonstration, was explored earlier by a group of educational researchers: Sanger, Phelps, and Feinhold (2000). Specifically, the authors studied whether students' conceptual understanding of what happens at the molecular level when a heated and sealed can containing water is cooled is improved when a specially designed technology is added to traditional teaching approaches. Introductory chemistry classes at a small mid-western university were enlisted to answer this question. Seventy second-semester students were part of a control group where a static chalkboard drawing based on kinetic-molecular theory and a can-crushing demonstration were used in instruction. Eighty-six first-semester students were part of an experimental group that used a computer animation in addition to the instruction given to the second-semester students. Then, the same quiz was given to both sets of students in the next class following the experimental instruction. After qualitative and quantitative analysis, the researchers discovered that students exposed to computer animations of molecular-level chemical processes were more successful at answering conceptual questions about particulate phenomena. These students were also less likely to simply quote equations in their response to questions.
Technology and Modeling of Gas Behavior
Continuing with technology use in the classroom, Snir, Smith, and Raz (2003) looked at the effectiveness of computer software in teaching students about particulate models, the meaning and use of models, and the structure of matter.
The research examined nine fifth-grade and sixth-grade students from an urban U.S. public school and two seventh-grade classes in rural Israel. These students were given several problems to consider that a particle nature of matter model could solve, while competing ideas and theories could not. For example, when two beakers containing equal volumes of chemicals are added, the resulting volume would be expected to equal twice the individual volumes. This is not always the case, as when water and alcohol are mixed the combined volume is less than two. A particulate nature of matter model can demonstrate the reason for the resulting volume. The software used by the authors provided visual and textual information to help students grasp essential ideas. After instruction and software use, group pre-tests and post-tests were given, followed by qualitative analysis.
The research team discovered that, by using a software tool and carefully designed instruction, middle-school students can comprehend the meaning and use of models in explaining scientific phenomena. Explanatory models can be a powerful tool for promoting student understanding if the model used for teaching can be readily grasped by the students, and if students learn the practical value of models in real-world science endeavors. Also, the explanatory nature of scientific models can be understood at a deeper level by students with strong macroscopic views of matter.
Discussion
So, what do these studies involving middle-school and college students show, in general. about students' ability to learn about and explain the invisible?
First, helping students to "see" the invisible is not an impossible task, nor even an exceptionally difficult one. Students showed improvement with even relatively unsophisticated instruction methods. For instance, Singer et al. (2003) employed real-world settings to provide perspective and to situate the invisible, but familiar, subject of air and air pollution. The urban school students who participated in their study should have been especially familiar with poor air quality. Engaging students in group and classroom discussions about their experience with clean and dirty air, and what they expected are the disadvantages of the latter, would be a good "grounding point" for introducing this topic that directly impacts their lives. Securing the invisible-to-personal experience helps make the abstract more concrete. If students are unsure about whether or not they have personal experience seeing the invisible, they can be reminded that the invisible can be detected in non-visual ways, like through smell. A brief walk around the school or local community can also help bring the issue of the invisible to life (industrial or motor vehicle odors, discoloration of buildings and plants) (Singer et al., 2003).
Once the setting has been established, content or structure can be added with more certainty that it will stand. Singer et al. (2003) referred to this addition as the "content phase" of their instructional sequence. This phase is conducive to the inclusion of technology -- both physical and computational models and computer animation (Sanger et al., 2000; Singer et al., 2003) -- and incorporation of techniques, such as explanatory modeling, used by practicing scientists (Snir et al., 2003).
Probably each of the research authors would agree that more effort must be focused on students' qualitative understanding of the invisible world. Educational research indicates that students can successfully solve algorithmic chemistry problems without having an appropriate comprehension of chemistry principles. Thus, as necessary as quantitative understanding is for fully understanding the microcosm, the qualitative meaning of formula components, operations, and results must be stressed to students.
Looking at another foundational issue, knowledge and adept use of inscriptions (diagrams, figures, tables, graphs, photographs, models, and formulas) and inscription production can greatly enhance communication among students and teachers and ultimately increase the understanding of molecular states and behavior. This conclusion is implied from the studies examined in this paper. Inscription use and quantitative/ qualitative sense-making help students make the invisible visible.
Finally, this collection of research on the invisible realm supports a "constructivist" approach to educating students on this complex topic. Students' present knowledge state on molecules would need to be assessed first. Then, appropriate cognitive-level instructional tools can be supplied to students so they may construct their own understanding of the microcosm with the aid of the teacher and fellow students. The instructor facilitates students' skillful personal and collective use of the tools; thus, students may make significant new connections from their own knowledge base and experience to a meaningful, scientific cognition of the particulate model of matter.
Conclusion
From grade school to graduate school, the nature and behavior of molecules is a difficult environmental-science concept to make clear to students. Traditional classroom lectures, textbook readings, and assignments are typically insufficient for instilling a deep understanding of microscopic particle concepts in students. Revised teaching methods, including instructional tool use like computer-generated images, have been shown to improve students' comprehension of the world of invisible particles.
The examined research on instructional tool use can help traditional classroom instructors identify demonstrated improvements for their own environmental-science teaching. Furthermore, this research shows that helping students to see the invisible is not an overwhelming challenge. Rather, the results of the research indicated that, with the proper initiation, content, application, and sequencing of learning material, students can achieve a deeper understanding of the microscopic world. Thus, students may discover a world they have been living in, but of which they have been largely unaware.
References:
- Sanger, M. J., Phelps, A. J., & Feinhold, J. (2000). "Using a computer animation to improve students' conceptual understanding of a can-crushing demonstration." Journal of Chemical Education, 77(11), 1517-1520.
- Singer, J.E., Tal, R., & Wu, H.-K., (2003). "Students' understanding of the particulate nature of matter." School Science and Mathematics, 103(1), 28-44.
- Snir, J., Smith, C. L., & Raz, G. (2003). "Linking phenomena with competing underlying models: A software tool for introducing students to the particulate model of matter." Science Education, 87, 794-830.
This article originally appeared in the 09/01/2005 issue of Environmental Protection.
About the Author
Anthony J. (Tony) Sadar, CCM is a certified consulting meteorologist (CCM), founder of Environmental Science Communication, LLC, of Pittsburgh, Pa., and co-author of Environmental Risk Communication: Principles and Practices for Industry (CRC Press/Lewis Publishers, 2000). Sadar holds graduate degrees in both environmental science and science education.